Science certainly hasn’t identified every useful chemical on the planet, yet discovering natural substances that have valuable healthcare applications is labor-intensive. The search can involve things like expeditions to the Amazon or painstakingly culturing rare microbes gathered from ocean deeps. And useful results can take decades.
Instead, techniques of synthetic biology let researchers design new molecules from scratch that have the attributes they seek. They can then synthesize them chemically or induce fast-growing organisms like bacteria or algae to produce them. This approach has already yielded promising new therapeutics that may relieve some formerly undruggable conditions.
A whiff of virus protection
What if you could inhale a few puffs of nasal spray to protect yourself against COVID-19? That’s the promise of research from the University of Helsinki. If it makes it through the rigorous pipeline from lab to drug, the molecule currently known as TriSb92, sniffed before exposure, could keep the virus from taking hold in the nasal membranes.
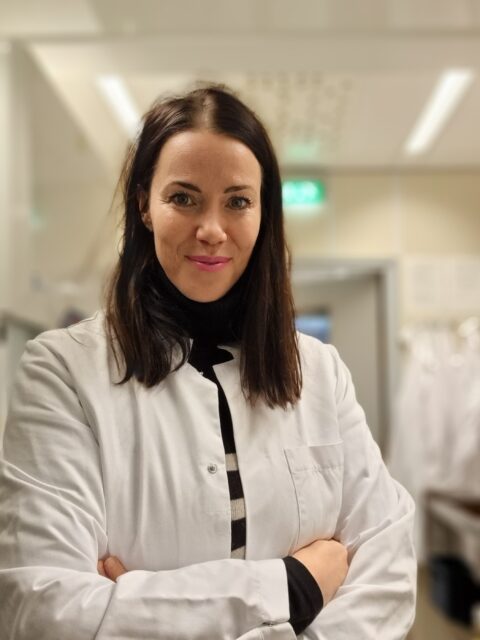
TriSb92 isn’t found in nature; the molecule was created in a lab.
The Helsinki team wanted to discover a molecule that would bind to an area within the COVID-19 spike protein that doesn’t change when the virus mutates.
“Typically, these structures—virus’ Achilles heels—are pivotal to the viral life cycle and transmission, so they don’t vary when the virus mutates. This way, the members of an entire virus family can be blocked by one solution,” says Anna R. Mäkelä, the lead researcher.
They used a new system for discovering biological drugs called Sherpabodies. These are fragments of a human protein that have been modified to include random amino acid sequences that can bind with other molecules.
The Helsinki team was looking for Sherpabodies containing sequences that would bind with that unchanging COVID-19 spike protein. They discovered 15 Sherpabody binders and developed the one with the strongest action, making it even stronger by connecting three copies of the molecule together. The result, TriSb92, bound with all the extant variants of COVID-19, including Omicron, neutralizing them by attaching to that viral Achilles heel.
In tests with mice, TriSb92 protected mice from contracting the virus when they’d inhaled it as long as eight hours before exposure and up to eight hours after exposure. This means that the molecule has the potential to treat early-stage COVID-19, as well, by keeping the virus from proliferating in the nasal cavity.
TriSb92 also neutralized a new coronavirus that was just discovered in a Laos bat cave. Mäkelä thinks it is also likely to be equally effective against new COVID-19 variants, as well as against related viruses that may evolve in nature.
Mixing biology with chemistry
Synthetic biology got its start in 2002, when biologists recreated the polio virus, according to the National Human Genome Research Institute. In gene editing, scientists insert or remove a bit of DNA into an organism’s genome. In synthetic biology, they insert larger pieces of DNA that may come from other organisms or be completely novel.
The first International Meeting on Synthetic Biology took place at MIT in 2004, creating interest in the field from engineers, biologists, chemists, physicists and computer scientists. The year 2013 marked the first time synthetic biology produced a commercial product: artemisinin, a drug for malaria, produced by re-engineered yeast.
Today, increases in computing power, advanced laboratory methods and intense interest from investors fuel a thriving ecosystem for research and startups.
Recently, a team of synthetic chemists and synthetic biologists at the University of California, Berkeley, and the Lawrence Berkeley National Laboratory invented a way to get E. coli, the intestinal bacterium that’s the workhorse of genetic engineering, to produce a molecule not found in nature, paving the way for bio foundries for a broad range of new chemicals.
Organisms have ways they actively bring molecules into cells, whether those are nutrients, hormones or cofactors. We coopted that system to bring the unnatural molecule into the cell, where it gets incorporated into the protein at the right place.
—John F. Hartwig, lead researcher, UC Berkeley
They started with E. coli that had been engineered to produce limonene, a component of citrus oil. First, they swapped one iron atom in a natural protein for an atom of iridium. This protein is a cofactor—a chemical compound that assists in enzyme activity. Then, they bathed the bacteria in a nutrient bath that included this manmade molecule. The bacteria absorbed the molecule and incorporated it into themselves.
Lead researcher John F. Hartwig from UC Berkeley explains, “Organisms have ways they actively bring molecules into cells, whether those are nutrients, hormones or cofactors. We coopted that system to bring the unnatural molecule into the cell, where it gets incorporated into the protein at the right place.”
Changing this enzyme caused the bacteria to produce a different form of limonene that included the altered molecule. As the bacteria reproduce, this novel molecule gets passed along, and the bacterial culture continues to produce it.
This research blurs the line between chemistry and biology, Hartwig says, and increases the range of chemicals that yeast and bacteria could make.

Chemical reactions in natural systems are complicated and difficult to reproduce in the lab, Hartwig notes, while there are simpler molecules that can be made in the lab but cells can’t make. The vast majority of drugs could never be made by a natural system.
“The vision is to have nature produce those molecules we make in the laboratory at the same level of efficiency and sustainability as natural products are made,” Hartwig says. “Just feed cells some glucose and amino acids, and maybe something synthetic, and out of that cell comes this complicated molecule that would have a great function in biology or medicine.”
This approach could also be used to modify natural substances to make them less toxic or more useful as a drug.
New targets for therapeutics
Instead of testing natural molecules against various pathogens or biological processes to see their effects, synthetic biologists start with the biological process they want to improve. Then, using a combination of computation, biology and chemistry, they custom-design molecules to fit.
For example, Crohn’s Disease causes inflammation of the digestive tract. Enterome, a biopharmaceutical company, found that Crohn’s patients had high levels of FimH, an inflammatory chemical produced by some bacteria in the gut. It created Sibofimloc, a molecule that blocks these bacteria from adhering to the gut wall and releasing FimH. The drug is in phase 2 clinical trials to see if it can relieve Crohn’s.
Athira Pharma completed its phase 2 clinical trial for a new molecule to treat early-stage Alzheimer’s disease, dementia and Parkinson’s disease dementia. Fosgonimeton is an engineered molecule that can be injected under the skin to penetrate the brain, where it may boost the action of a chemical that stimulates the growth of new blood vessels. This could reduce loss of brain cells and even regenerate brain tissue, leading to improvement in memory and brain function.
Insilico Medicine used artificial intelligence to identify a new path for treating the orphan disease idiopathic pulmonary fibrosis, a lung disease due to scarring of lung tissue. Then, AI designed ISM001-055, a molecule to improve the scarring. The drug just entered the first phase of clinical trials.
Chemistry, biology or computation alone could never achieve these potential breakthroughs.
Says UC Berkeley’s Hartwig, “We’re making biology do laboratory chemistry and giving it some tools it wouldn’t have naturally.”
In other words, synthetic biology can extend our definition of “natural” in ways Carolus Linnaeus could never have imagined.
Lead image by Benjamin Lehman/Unsplash